Exploring the Fundamentals of Qubits in Quantum Computing
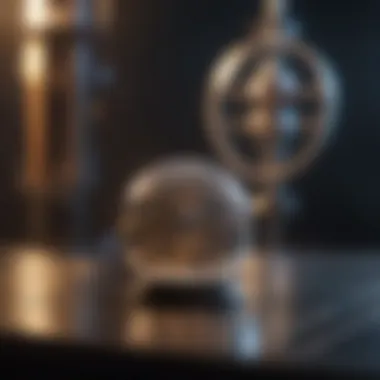
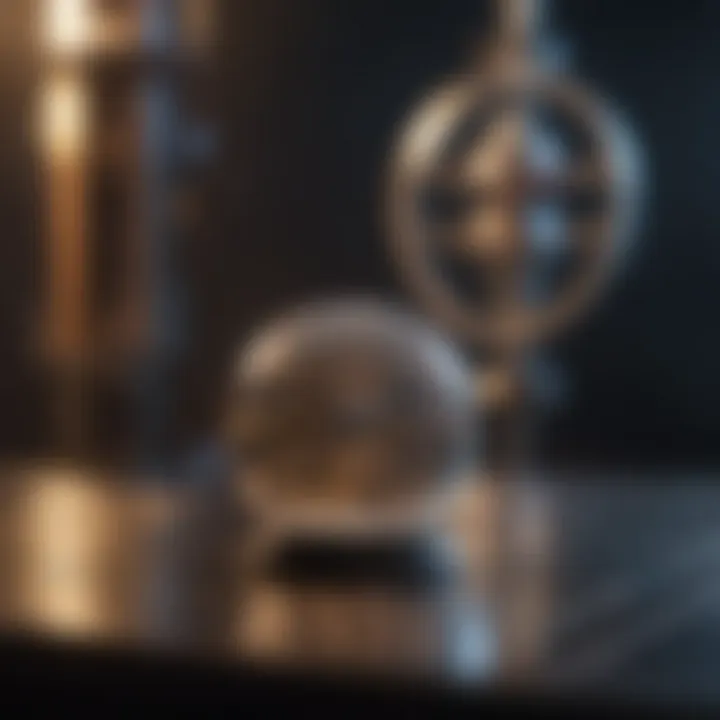
Intro
In the realm of quantum computing, a shift in how we perceive and process information is taking shape. At the heart of this transformation lies the concept of qubits—the fundamental units that enable quantum computers to outperform classical systems in certain tasks. But what exactly is a qubit? How does it differ from the familiar bits we’ve known for decades? This article invites you to explore these questions and more, revealing the intricate nature of qubits and their significant role in the future of technology.
As we step into the world of quantum mechanics, it's essential to clarify some foundational terms that will frame our understanding. By grasping these concepts, both novices and seasoned enthusiasts can navigate the complexities of this cutting-edge field more effectively. This journey will uncover different types of qubits, their various implementations, and the challenges faced by researchers as they develop this burgeoning technology.
Get ready to dive deep into the quantum world, where the rules differ from anything you've ever encountered. From the simplest descriptions to intricate algorithms, the path ahead is filled with fascinating insights that excite the mind and broaden our comprehension of computation itself.
Prologue to Quantum Computing
In recent years, the field of quantum computing has garnered significant attention, not just from scientific circles but also from various sectors eager to harness its potential. This interest stems from a need to solve complex problems that remain challenging or even infeasible for classical computers. As we start this exploration into quantum computing, it is essential to understand the foundation it rests upon: quantum bits, or qubits.
Quantum computing holds the promise of revolutionizing numerous industries—from cryptography to pharmaceuticals—giving rise to new paradigms of computation that traditional systems simply cannot match. With the ability to process vast amounts of data at unprecedented speeds, the implications of quantum computing extend far beyond mere computational advantages; they propose a shift in how we perceive and apply technology in our daily lives.
Historical Context of Quantum Computing
Going back in time, the concept of quantum computing emerged from the theoretical frameworks laid by physicists in the early 1980s. Richard Feynman and David Deutsch were pivotal figures, laying the groundwork and proposing the notion that quantum mechanics could inform computational processes. Their vision was not merely theoretical; it was about pushing the boundaries of computation into the very realm of the atomic and subatomic particles.
The early work centered around understanding how quantum mechanics worked under the hood. Unlike classical systems, which rely on bits as fundamental units of data, quantum systems utilize qubits, which harness the principles of superposition and entanglement. This shift from classical to quantum lays the groundwork for a reimagined computing landscape.
Years of research culminated in the 1990s when notable algorithms such as Shor’s and Grover’s began demonstrating practical applications of quantum principles to tackle problems like integer factorization and database search. These developments sparked a frenzy of interest in quantum computing, leading to serious investment and serious consideration from both academic and corporate spheres.
The Need for Quantum Computing
In an age defined by digital transformation, the limitations of existing computational systems are becoming more apparent. Classical computers rely on binary data strings—0s and 1s—and operate in predictable, linear manners. As technology advances, the complexity of problems has increased, demanding solutions that go far beyond classical capabilities. The need for quantum computing arises from various fronts:
- Data Size: With the explosion of big data, analyzing extensive datasets quickly and efficiently is critical. Classical systems struggle with this.
- Computational Power: Tasks like optimization and simulation, particularly in fields like logistics, finance, and material science, benefit greatly from quantum applications.
- Cryptography: The rise of quantum computing presents both a threat and an opportunity; while some encryption methods may be broken using quantum algorithms, new quantum encryption methods can enhance security.
Understanding these needs is fundamental to grasping the importance of qubits in quantum computing. They represent a new frontier in processing information, pushing us toward innovative solutions that can address today’s and tomorrow's challenges.
"Quantum computing isn't just a step forward; it's a leap into a realm of possibilities where complexity and speed no longer exist in isolation."
As we proceed through the article, we will examine the relationship between qubits and their classical counterparts, dive into the mathematical underpinnings of qubits, and explore their unique properties that facilitate revolutionary breakthroughs in computation.
Defining the Qubit
In the realm of quantum computing, the qubit stands as a pivotal concept—a step beyond the classical bit that undergirds modern computing systems. Understanding qubits is fundamental for anyone keen on grasping how quantum computing operates and its potential to revolutionize various sectors. This section elucidates the nature of qubits, exploring their distinctive features and mathematical foundations, which differentiate them from classic bits.
Qubit vs. Classical Bit
At the heart of computing lies the bit, the simplest form of data. In traditional computing, a classical bit can only exist in one of two states: 0 or 1. These binary states are the building blocks for data processing and storage. In contrast, a qubit, or quantum bit, operates on a different principle. It can also represent 0 or 1, but here's the kicker: it can exist in a state of both 0 and 1 simultaneously, thanks to a phenomenon known as superposition.
This property allows quantum systems to process vast amounts of information in parallel. Imagine a qubit like a spinning coin; while it's in motion, it's neither just heads nor just tails—it's a mixture of both. This potential makes qubits extraordinarily potent for complex computations.
Key Differences between Qubits and Classical Bits:
- States: Classical bits are either 0 or 1, while qubits can be in a superposition of both.
- Information Capacity: A single qubit can hold more information than a classical bit because of its ability to represent numerous states at once.
- Entanglement: Qubits can be entangled, meaning the state of one qubit can depend on another, irrespective of the distance between them. This doesn’t happen with classical bits.
"In the world of quantum mechanics, what appears strange at first often holds the secrets of profound capabilities."
Mathematical Representation of Qubits
The mathematical backbone of qubits falls predominantly within the framework of linear algebra. A qubit can be mathematically represented as a linear combination of its basis states. Formally, this is expressed as:
[ | extQubit \rangle = \alpha |0\rangle + \beta |1\rangle ]
Where:
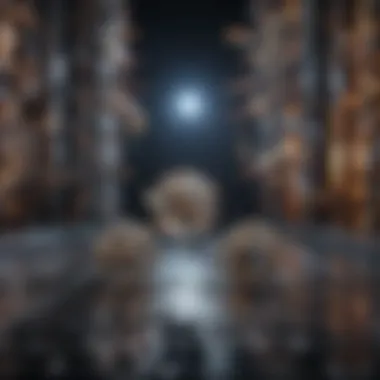
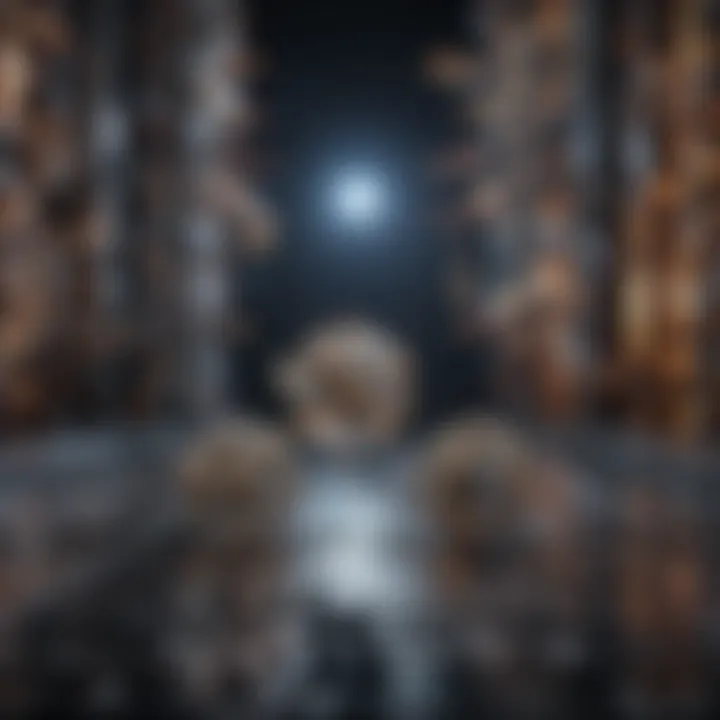
- |0⟩ and |1⟩ represent the basis states of the qubit.
- α and β are complex numbers that describe the probability amplitudes of the respective states, where ( |\alpha|^2 + |\beta|^2 = 1 ). This ensures the total probability remains one, a critical aspect of quantum mechanics.
The elegance of this representation illustrates the qubit's ability to exist in various states, which is the cornerstone for quantum gates and algorithms.
In essence, knowing how to represent qubits mathematically not only deepens our understanding of quantum systems but also lays the groundwork for developing quantum algorithms that harness this unique behavior to solve problems far beyond the reach of classical computing.
Properties of Qubits
The properties of qubits are crucial in understanding why these units of quantum information can revolutionize computing as we know it. Unlike classical bits that reside in the familiar binary state of 0 or 1, qubits wield remarkable properties that allow them to perform computations in ways that today’s technology simply cannot match.
Superposition Explained
At the heart of quantum mechanics is superposition. This principle allows qubits to exist in multiple states simultaneously – think of it as taking an ordinary coin, flipping it, and having it land on both heads and tails at the same time. In binary terms, a qubit can represent not just a 0 or a 1, but both at once. This enables quantum computers to handle vast amounts of information in parallel, providing significant performance improvements over classical systems.
When a qubit is in superposition, computations can be performed on numerous possibilities at once. For example, if you have four classical bits, they can represent one of 16 combinations at any time. However, four qubits can represent all 16 combinations simultaneously. This ability leads to exponential growth in computational power, potentially solving complex problems much faster than traditional methods.
Entanglement and its Significance
Next comes entanglement, a phenomenon that makes quantum computing nothing short of a game changer. When qubits become entangled, the state of one qubit becomes dependent on the state of another, no matter the distance between them. Imagine you have two dice that are magically linked; rolling one will instantaneously change the outcome of the other, regardless of how far apart they are.
This opens doors for powerful computing scenarios. Entangled qubits can transmit information in a way that fundamentally enhances the processing capabilities of quantum algorithms. The magic lies in the fact that changes to one qubit appear to instantly affect its entangled partner, allowing for quick and efficient data manipulation, already showing promise in fields like cryptography and optimization.
Measurement of Qubits
Finally, we must delve into the measurement of qubits. When we measure a qubit, it effectively 'chooses' a state between its potential values – collapsing the superposition into either a 0 or a 1. This action has profound implications. Measurement induces what quantum physicists term “wave function collapse.” Once measured, the qubit no longer holds its previous potential states, thus limiting the essence of superposition that makes quantum computing powerful.
This characteristic presents a unique challenge; measurements can introduce noise and imperfections to computations, requiring robust error correction methods to mitigate these effects. Understanding how and when to measure qubits is critical in ensuring reliable outcomes in quantum computations. As we move forward in quantum computing development, mastering the art of measurement will be as fundamental as the dynamics of superposition and entanglement.
"The tour de force of quantum computing lies in its qubits, where properties such as superposition and entanglement allow for unprecedented computational efficiency."
Overall, these properties make qubits not just fascinating components of quantum computing but essential pillars upon which this technology stands. Their unique qualities allow quantum systems to approach problems that were once deemed too complex for traditional computations.
Types of Qubits
Understanding the diverse types of qubits is crucial for grasping the potential and operational capabilities of quantum computing. Each qubit type comes with its own strengths and weaknesses, impacting performance, efficiency, and scalability of quantum systems. As the field continues to evolve, recognizing these differences allows investors and enthusiasts alike to comprehend how quantum technology might unfold and which avenues hold promise.
Superconducting Qubits
Superconducting qubits have become the poster child for quantum computing research. These qubits utilize superconducting circuits, which operate at extremely low temperatures. The main advantage here is that they allow for relatively fast operations, making them ideal for building quantum computers that can perform complex calculations in a shorter duration. The reasoning behind this speed is linked to their manipulation of microwave pulses to control the state of the qubit.
- Key Characteristics:
- Fast operation, around 10 ns
- Relatively easy to fabricate
- Coupling with microwave technology
A challenge that researchers face with superconducting qubits is decoherence, which refers to the loss of information due to environmental interactions. Despite this hurdle, innovations in shielding and cooling techniques have led to improvements in performance and reliability. So, as many companies focus on expanding superconducting qubit technology, it is viewed as a significant player in the race for quantum supremacy.
Trapped Ion Qubits
Trapped ion qubits represent a different approach altogether. They leverage the quantum states of ions confined in electromagnetic fields. The unique aspect of trapped ions is their extreme coherence time, which makes them very stable. It’s not just about stability; they can also be precisely manipulated using laser technology. These properties grant trapped ions an edge in error correction, a vital component for functional quantum systems.
- Benefits of Trapped Ion Qubits:
- Long coherence times
- High fidelity in operations
- Scalable with additional ions
However, trapped ion systems can be labor-intensive to manage and require intricate setups. The operational precision means that any minor deviation can lead to errors in computation. Despite these challenges, the capabilities of trapped ion qubits suggest they could be crucial for future quantum algorithms, making them an exciting area for further exploration and investment.
Topological Qubits
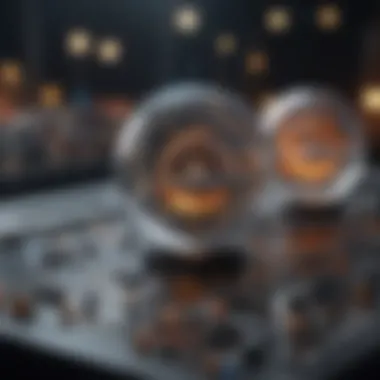
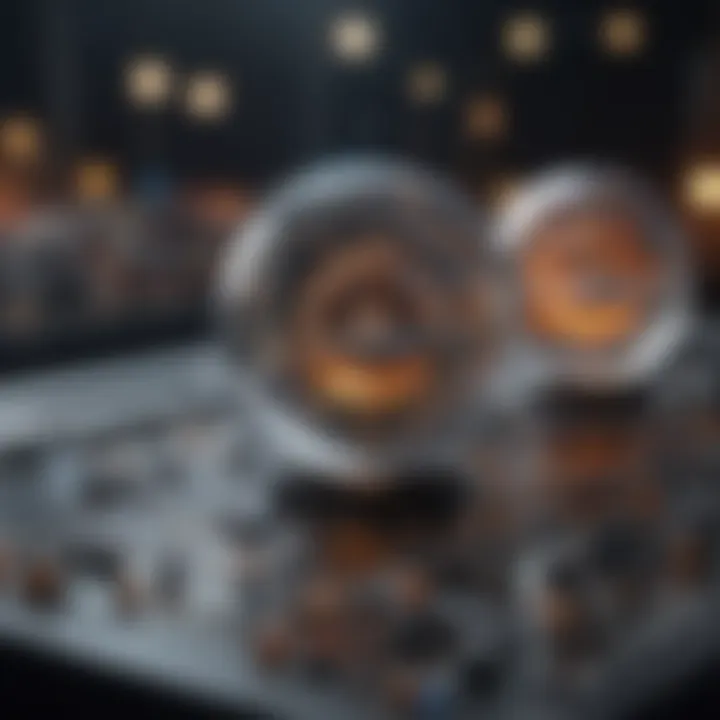
Topological qubits are a relatively new concept in the quantum landscape. These qubits rely on the principles of topology, which deal with properties that remain unchanged when a system undergoes continuous transformations. The focus on utilizing special particles known as anyons allows for robust qubits that are less susceptible to environmental noise. This resilience makes them ideal candidates for fault-tolerant quantum computing.
- Advantages of Topological Qubits:
- Increased error resistance
- Potential for scalable quantum computations
- Utilizes braiding processes for encoding information
Currently, the field of topological qubits is still in its infancy. Research is ongoing to understand their behavior better and figure out practical applications. The potential of these qubits, however, sparks a great deal of excitement among researchers and investors alike, as they may pave the way for a more robust and scalable quantum future.
End on Types of Qubits
Each type of qubit offers a unique contribution to the ongoing evolution of quantum computing. Superconducting qubits advocate speed, trapped ions excel in stability and accuracy, while topological qubits champion resilience against errors. Understanding these distinctions not only provides insight into the current state of quantum research but also helps in anticipating future advancements. As the landscape of qubit technology evolves, so too will its implications for industries and investors keen on leveraging quantum capabilities.
Qubits in Quantum Algorithms
Quantum algorithms are the beating heart of quantum computing, and qubits are the vital organs that enable them to function. Without these quantum bits, the promise of exponentially faster computation—a key advantage of quantum mechanics over classical methods—would be just a pipedream. In this section, we explore the nuances of how qubits contribute to the effectiveness of quantum algorithms and their unparalleled benefits in problem-solving capabilities.
Role of Qubits in Quantum Gates
At the core of quantum computation is the concept of quantum gates, essential elements responsible for manipulating qubits. Much like classical logic gates that govern the behavior of traditional bits, quantum gates perform operations on qubits in ways that allow them to retain and manage their unique properties. The most notable is how they can exist in a state of superposition.
These operations do not merely toggle values from 0 to 1; they can create complex states representing multiple values simultaneously. For instance, a Hadamard gate can set a qubit into a superposition state, allowing it to embody both 0 and 1 at the same time. This characteristic is what gives quantum computers their extraordinary speed and efficiency, allowing them to compute vast possibilities at once.
In this way, qubits encoded to represent more than one binary state can execute multiple calculations in parallel. So when we talk about algorithms, it’s crucial to grasp that the manipulation of qubits directly dictates the efficiency and outcomes of quantum processes.
Quantum Algorithms Utilizing Qubits
As the technology matures, several quantum algorithms have garnered attention for their revolutionary potential, capitalizing on the unique properties of qubits. Algorithms like Shor’s and Grover’s demonstrate how qubits can tackle problems that would be infeasible for classical computers.
- Shor’s Algorithm: This algorithm takes prime factorization to a whole new level, exploiting the superposition of qubits to find factors of large numbers in polynomial time, which classical algorithms struggle with. This capability poses significant implications for cryptography and the security of information systems based on current encryption standards.
- Grover’s Algorithm: Unlike its classical counterpart, which needs to check each potential solution individually, Grover’s employs qubits to conduct a search over unsorted databases with quadratic speed-up. This is particularly useful for algorithm optimization in various sectors, including finance and logistics.
The integration of qubits in such algorithms symbolizes a leap forward in computational technology, potentially transforming industries by enhancing capabilities of data processing, optimization, and simulation.
With the horizon of quantum computing ever-expanding, the role of qubits in various algorithms is not just an academic pursuit; it's the foundation of future progress and innovation. Their adaptability and power in manipulating data revolutionize what we think is possible with computation.
Challenges in Qubit Implementation
Understanding the challenges in qubit implementation is crucial for anyone keen on the future of quantum computing. While qubits promise incredible improvements over classical computing, the road to harnessing their full potential is riddled with obstacles that researchers and engineers need to navigate. These challenges can impact the reliability, scalability, and practical application of quantum devices.
Decoherence Issues
Decoherence is one of the primary concerns in the realm of quantum computing. It refers to the loss of quantum coherence, meaning that qubits, which should exist in a state of superposition, begin to lose their quantum properties due to environmental interactions. Essentially, decoherence leads to a situation where the information contained in a qubit is lost.
The fragility of qubits is akin to trying to balance a house of cards during a windstorm; any slight disturbance can topple their quantum state. This can significantly degrade the performance of quantum algorithms.
To mitigate decoherence, researchers are exploring various approaches such as error correction techniques and developing materials that exhibit better coherence properties. For instance, superconducting qubits are designed to operate at very low temperatures to reduce thermal noise, making them less susceptible to decoherence.
"Addressing decoherence is not just about making better qubits; it’s about reconciling quantum mechanics with the practical limits imposed by our environment."
Scalability Challenges
Scalability presents another formidable challenge in qubit technology. Currently, many quantum computers operate with a limited number of qubits due to the constraints of existing technology, leading to a bottleneck in performance. To realize practical quantum computers, thousands, if not millions, of qubits may be necessary. However, scaling up qubit systems introduces a host of difficulties.
For instance, when trying to increase the number of qubits, consistency and connectivity among them must be maintained. The more qubits you have, the more complex the interactions become. The intricate wiring needed to control these qubits can become unwieldy and prone to error. Hence, researchers are investigating different architectures, such as quantum annealers or modular systems, to enable more effective scaling.
In essence, resolving scalability issues may determine the overall success of quantum computing in real-world applications. Embracing innovative designs and methodologies holds the key to overcoming these hurdles, ensuring a future where quantum computers are not confined to the laboratory but become instrumental in various industries.
Advancements in Qubit Technology
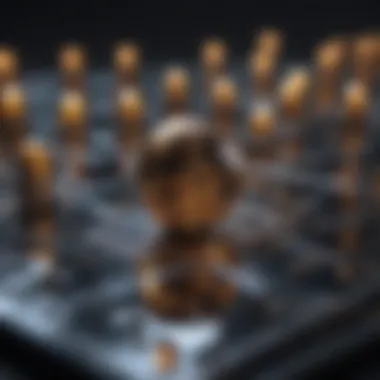
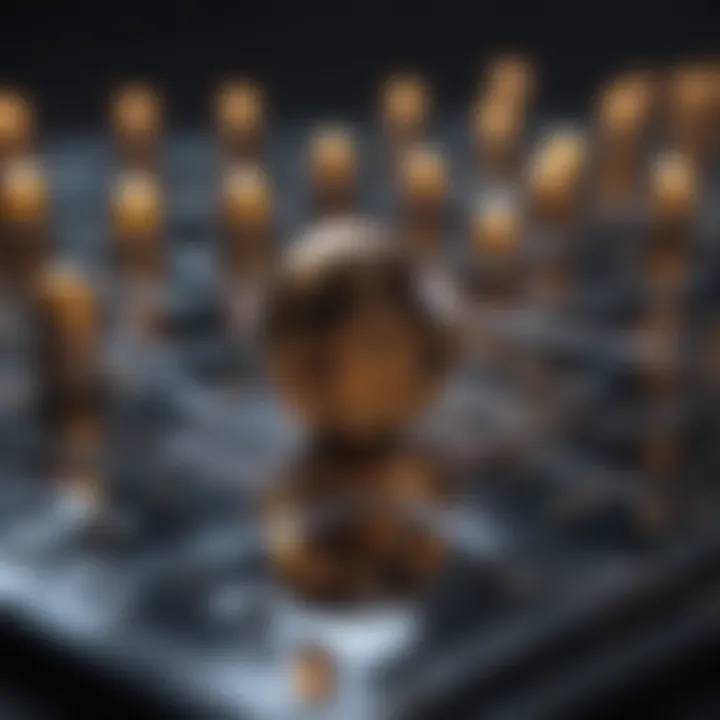
The realm of quantum computing relies heavily on the evolution of qubit technology. As we explore this intricate domain, understanding the advancements in qubit technology is paramount. It informs not only the efficiency of quantum computations but also lays the groundwork for practical implementations in various fields, from cryptography to drug discovery.
Recent Innovations
In recent years, innovations in qubit technology have made waves. Researchers and technologists have been relentless in their pursuit of enhanced qubit designs that can keep pace with the growing demands of quantum algorithms. Some notable advancements include:
- Superconducting Qubits: Companies like IBM and Google have fine-tuned superconducting qubits, allowing for longer coherence times. This means these qubits can maintain their quantum state longer than before, crucial for complex calculations.
- Trapped Ions: IonQ and Honeywell are pushing the boundaries with trapped ion qubit systems. Recent advancements allow for scalable configurations that significantly improve accuracy and reduce error rates in quantum computations.
- Photonic Qubits: Another frontier is the use of photons, which are being harnessed for qubits. Startups like Xanadu are pioneering photonic quantum computing, where qubits are represented by light particles, enabling faster and more efficient processing.
These innovations not only demonstrate the remarkable progress in the field but also hint at future possibilities. The continuous improvement in error correction protocols has also played a critical role.
"The pathway to scalable quantum systems hinges on the enhancement of qubit performance and error resilience," states a lead researcher in quantum technology at MIT.
Future Directions and Research Trends
Looking ahead, several trends are emerging that could shape the future of qubit technology. Investors and enthusiasts should pay attention to:
- Hybrid Quantum Systems: The integration of different qubit types, such as superconducting and topological qubits, could lead to more robust quantum processors with improved capabilities.
- Materials Innovation: The search for new materials that can sustain quantum states is ongoing. Research into materials like graphene or diamond could unlock more efficient qubit designs.
- Quantum Networking: As quantum computers become more commonplace, the need for interconnected systems is rising. Research is focusing on developing quantum networks that could exceed classical capabilities in communication security.
In summary, the advancements in qubit technology are not just about refining existing designs but are also paving the way for new paradigms in computation. Keeping an eye on these trends will be vital for understanding how to harness the full potential of quantum computing in real-world applications.
The Future of Quantum Computing
The horizon of quantum computing holds a plethora of possibilities that not only promise to reshape how we process information but also influence various facets of our everyday lives. As we explore the future of quantum computing, it's essential to recognize that the fruits of this discipline are still ripening. There's a palpable excitement among researchers and industries alike as they venture further into this uncharted territory.
The fundamental shift towards quantum paradigms arises from the limitations of classical computing, particularly as we face increasingly complex problems. Problems that seem insurmountable today may very well become solvable through the power of qubits. Those tiny units of quantum information, with their unique traits, herald a revolutionary change that could extend to diverse sectors, from healthcare to climate modeling and beyond.
Potential Applications and Impact
The potential applications of quantum computing are vast and varied. To provide a clearer picture, consider the following areas where quantum technologies could lead the charge:
- Drug Discovery: Quantum computing can simulate molecular interactions at a level classical computers can only dream of, leading to faster drug development cycles. Imagine pharmaceutical companies being able to analyze millions of chemical compounds in mere minutes!
- Cryptography: The strength of current systems hinges upon the complexity of factorization. Yet, quantum algorithms threaten to crack these systems wide open, propelling us to create more robust, quantum-resistant encryption methods.
- Optimization Problems: Industries like logistics or finance often wrestle with optimization problems. Quantum computing's ability to process and analyze vast datasets more efficiently could revolutionize how we manage supply chains or stock portfolios.
- Artificial Intelligence: Machine learning could see an exponential boost. With faster data processing capabilities, AI systems could learn and adapt quicker, making them more effective at decision-making.
The impact of these advancements is not merely theoretical. Companies across various sectors are already investing heavily in quantum research, indicating a recognition of its potential long-term benefits. Such investments signal a seismic shift in the landscape of technology that can redefine competitive advantages.
Influence on Financial Sector
As markets become more interconnected and complex, the financial sector stands to gain immensely from quantum computing. Here’s how:
- Risk Assessment: With the ability to analyze risk factors in real-time, financial institutions could better prepare for market fluctuations. Advanced algorithms capable of evaluating multiple scenarios could lead to smarter investment strategies.
- Portfolio Optimization: Imagine having an algorithm that assesses thousands of investment combinations—a task that would take a classical computer an eternity. Quantum computing offers this potential, thus allowing for optimized portfolios tailored to various risk appetites.
- High-Frequency Trading: Speed is king in high-frequency trading. Quantum computers can process vast amounts of data simultaneously, enabling traders to make split-second decisions based on the latest market movements.
- Fraud Detection: The capability to analyze transaction patterns more intricately could lead to improved detection of fraudulent activities, safeguarding institutions and their customers from potential breaches.
In essence, the implications of quantum computing in the financial sector could not only streamline operations but also usher in an era of unprecedented efficiency. Investing in this technology could very well become the differentiating factor that propels some firms ahead of their competitors.
"As we stand on the brink of a new technological paradigm that challenges classical notions of computation, understanding its implications becomes not just a scientific endeavor, but a necessary step towards adaptation in a rapidly changing world."
The End
As we bring our exploration of qubits to a close, it's essential to recognize the significant role this concept plays in the realm of quantum computing. Qubits, through their unique properties such as superposition and entanglement, form the backbone of this revolutionary technology. Rather than merely acting as a binary unit of information like classical bits, qubits offer a complex and rich system capable of parallel processing. This distinction is not simply academic; it holds substantial practical consequences.
Summary of Key Concepts
Reflecting on the discussions throughout this article, several core concepts stand out. First, the understanding of how qubits differ from classical bits is fundamental. Classical bits are locked in a state of 0 or 1, whereas qubits can exist in multiple states at once, a principle that allows quantum computers to handle vast amounts of data simultaneously.
Next, we highlighted various types of qubits, such as superconducting and trapped ion qubits, each with their own advantages and shortcomings. The differenecs inform their application in real-world quantum computing environments and set the stage for future development in processing power.
Furthermore, we touched on the practical applications of qubits in quantum algorithms; they are not just theoretical constructs, but rather integral components of technologies that can change game in fields like cryptography, optimization, and drug discovery. As the research into quantum computing advances, the inherent challenges we discussed—like decoherence and scalability—remain areas of vibrant investigation. These factors will undoubtedly influence how qubits are utilized and how effectively quantum computers can be implemented across various industries.
Implications for Investors
For investors keeping a keen eye on technological advancements, the evolution of qubit technology presses forward alongside burgeoning interest in quantum computing applications. Understanding these elements can offer unique insights into prospective investments. Quantum computing can potentially revolutionize industries ranging from finance to healthcare.
Moreover, as companies race to develop practical quantum solutions, those that meaningfully incorporate qubit technology stand to gain substantial market advantages. Investment in this area is not without risk, but the potential rewards are significant, as breakthroughs in quantum algorithms could lead to efficiencies and solutions that were previously unimaginable.
In summary, recognizing the nuances of qubits isn't just academic; it's a window into the future of technology and investment opportunities. As the landscape evolves, remaining informed will be crucial for any investor hoping to navigate the shifting tides of innovation.